Regime shifts
Regime shifts are large, abrupt, persistence changes in the function and structure of systems (Biggs et al. 2009). They have been documented in a wide range of systems including financial markets, climate, the brain, social networks and ecosystems. Regime shifts in ecosystems are policy relevant because they can affect the flow of ecosystem services that societies rely upon, they are very difficult to predict and often hard to impossible to reverse (Rocha et al. 2012).
These critical transitions have been documented on a broad range of marine, terrestrial and polar ecosystems (Scheffer 2009, Scheffer et al. 2001). Two well studied examples include the eutrophication of lakes, when they turn from clear to murky water affecting fishing productivity and on extreme cases human health (Carpenter 2003); or the transition of coral reefs from coral dominated to macro-algae dominated reefs, loosing the provision of services related to tourism, coastal protection and food (Bellwood et al. 2004). Controversial examples include dryland degradation or the shift from forest to savannas, where we know they can happen but we do not fully understand their mechanisms and there are disagreements about their drivers. Proposed examples of regime shifts includes the weakening of the Indian Monsoon or the weakening of the Thermohaline circulation in the ocean. We know both can happen from paleological records, but to what extent they are likely under present conditions is less understood.
What these phenomena have in common is that their scientific understanding relies on the same mathematical theory of dynamic systems. The behavior of a system can be described by a set of equations that define all possible values of their variables, for instance coral cover or fish abundance. These systems tend to fluctuate around regions of the parameter space that are called equilibrium or basins of attraction. Systems prone to regime shifts have more than one domain of attraction; this means that roughly under the same parameter values they can suddenly shift from one domain to another when a critical threshold is crossed. Note that these domains of attraction or regimes are not punctuated equilibriums, they are dynamic, they fluctuate within the boundaries of the basin of attraction. Thus for example, a forest is never the same forest twice: the species composition, their abundance and distribution is always changing as the forest endures fires, hard winters, storms or pests outbreaks. Yet its identity can always be characterized as a forest. The same can be said of any regime.
However, a regime shift does not imply any change of an ecosystem, it has to affect the feedback structure of the system that maintain its stability and emergent properties; this is, a change that effects its identity (eg. Forest, coral reef, mangrove). Scientist when they refer to regime shifts often emphasize that they are abrupt and persistent changes. It worth noticing that the abruptness and persistence are relative to the dynamics defining the system’s identity. Back to the forest example, one generation of trees can last hundreds of years, then a change over the time span of a decade is abrupt from the forest perspective.
History of the concept and evidence
First notions of systems that can have multiple attractors date back to 1885 when Henri Poincaré first described bifurcations. However, only until late 1960s these mathematical developments were introduced to ecology when leading scientist debated about stability in ecosystems (Holling 1973, Lewontin 1969), which lead to the first theoretical developments, often models, of potential regime shifts in fisheries, disease outbreaks, and grazing – harvesting systems. These early developments were criticized by the lack of empirical evidence in the 1980s, but by early 2000s enough evidence have accumulated on systems such as coral reefs and lakes, validating the existence of regime shifts and igniting further research on their detection and management (Scheffer et al. 1001, Folke et al 2004, Scheffer and Carpenter 2003)). Nowadays there are still some differences on how the concept is used across disciplinary fields. For example, while oceanographers consider a regime shift a phenomenon on the scales of decades that has to be driven by climate, marine biologist focus on the time span of key species life cycles.
Evidence of regime shifts has accrued in the last two decades. It comprises empirical evidence when experimentation is possible, observational evidence from long-term time series data, paleological records from sediments and ice cores, as well as mathematical models that explore the possible mechanisms explaining such observations. Table 1 presents a summary of some regime shifts with impacts on society. More information about regime shifts can be found at the regime shifts database www.regimeshifts.org
Table 1. Examples of well-known regime shifts and their impacts on ecosystem services.
Regime shift | Regime A | Regime B | Impacts of shift from A to B | Evidence | Source of evidence |
---|---|---|---|---|---|
Freshwater eutrophication | Non-eutrophic | Eutrophic | Reduced access to recreation, reduced drinking water quality, risk of fish loss | Strong | Observations, experiments, models |
Bush encroachment | Open grassland | Closed woodland | Reduced grazing for cattle, reduced mobility, increased fuelwood | Medium | Observations, experiments, models |
Soil salinisation | High productivity | Low productivity | Yield declines, salt damage to infrastructure and ecosystems, contamination of drinking water | Strong | Observations, experiments, models |
Coral reef degradation | Diverse coral reef | Reef dominated by macro-algae | Reduced tourism, fisheries, biodiversity | Strong | Observations, experiments, models |
Coastal hypoxia | Non-hypoxic | Hypoxic | Fishery decline, loss of marine biodiversity, toxic algae | Strong | Observations, models |
River channel position | Old channel | New channel | Damage to trade and infrastructure | Strong | Observations, models |
Wet savanna- Dry savanna | Wet Savanna | Dry savanna or desert | Loss of productivity, yield declines, droughts/dry spells | Medium | Models |
Why are regime shifts hard to detect and costly to reverse?
Empirical and theoretical developments have shown that regime shifts are hard to detect and sometimes hard or impossible to reverse. Change in slow variables (e.g. temperature in coral reefs, nutrients accumulation in lakes) often shrinks the basin of attraction, making the system more susceptible to shifting when exposed to shock events (e.g. hurricanes, storms) or the action of external drivers. When the shift occur the strength of feedbacks change and some times new feedbacks mechanism come to place, making difficult to restore the original system state. This difficulty is often termed ‘hysteresis’; this mean that the critical tipping point at which the system flip from one regime to another is not the same at which the system is expected to flip back.
An illustrative example is eutrophication, the shift from clear to murky waters in lakes. The most common driver of eutrophication is the accumulation of nutrients such as nitrogen and phosphorous, although it has been reported that it can also by caused by climate change alone. Excess of nutrients in the water often come from the use of fertilizers when growing food. Nutrients are naturally present in the soil and in combination with other indirect drivers such as deforestation, nutrient inputs both natural and anthropogenic can accelerate when rain comes and wash the soils. Nutrients accumulate on lake sediments and are kept by rooted plants. But when a threshold of nutrients on the water is transpassed, microalgae growth explodes creating algae booms. These booms reduce light penetration to the bottom of the lake, which reduces the ability of rooted plants to survive. With less rooted plants the nutrients accumulated on sediments are resuspended on the water column, reinforcing the original cause of the algae blooms. This is a new reinforcing feedback in place that makes the regime shift hard to reverse. Figure 1 shows the structure of the system by summarizing the key feedbacks that maintain the clear water regime or the eutrophic state respectively.
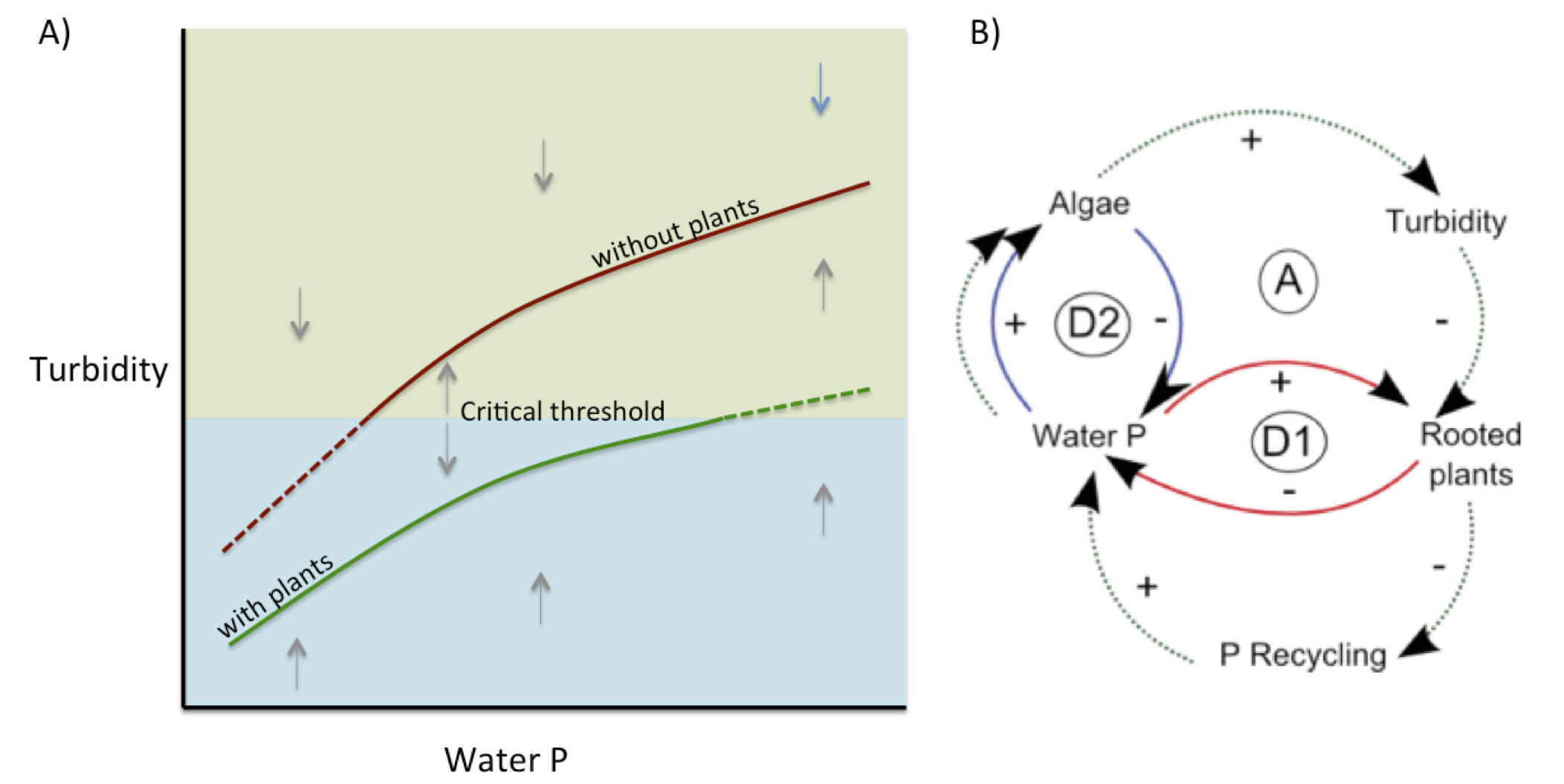
Detecting regime shifts is a challenging task. First one needs to clearly define the boundaries of the system and select focus variables that serve as indicators of the system state. Data requirements will depend directly on the spatial and temporal scales at which the underlying feedback mechanisms operate. Sometimes regime shifts are identifiable from historical data by looking at jumps on the time series; however statistical techniques are required to test whether it was a real regime shift. Amongst the most common test used are the principal component analysis, which compresses several time series of related variables into few uncorrelated ones; chronological clustering, sequential t-tests. Recent efforts on improving prediction rather than detection from past events have been focusing on the development of early warning signals (Scheffer et al 2012). They are based on the statistical signature of a system approaching a regime shift called critical slowing down which can be observed on the increased autocorrelation (temporal or spatial) and skewness while decrease in variance.
How to manage regime shifts?
Managing regime shifts implies both reversing the shift and avoiding them. In order to restore the system one has to manage the slow variables and bring them to a point well bellow the critical tipping point in order to broaden again the basin of attraction. In addition, active management is needed to weaken the feedbacks that keep the system locked on the undesirable regime while strengthening the feedbacks that will provide stability on the desirable one. Back to the example of eutrophication, lake management often requires reducing nutrient inputs to a level lower than originally required for the system to flip. This not only means reduction of fertilizers use, it also means locking nitrogen already present in the lake by using other substances such as iron to waken the nutrient recycling feedback. Other options include bio-manipulation, this is artificially increasing the abundance of species that will consume microalgae to avoid the likelihood of more algae blooms; or direct removal of sediments by dragging.
Improving resilience to regime shifts requires enhancing diversity. From the biological perspective it is often translated into functional groups, this is, set of species that perform more or less the same function on an ecosystem. On the eutrophication example, by increasing the abundance of species that feed on microalgae and reducing the species that feed on rooted plants, one can promote a shift back from a eutrophic state. Two important components of functional groups are response diversity and redundancy: while the former is the different responses that species have to environmental change, the later refers to the ability of certain species to replace others. But these basic notions of diversity are not only applicable to biological systems, one can think of diversity in society as an important component to build resilience to regime shifts. It translates to diversity of livelihoods, or different types of knowledge taken into account in decision-making.
Another important component to build resilience is spatial heterogeneity. The influence of drivers and some times feedback strength is not homogeneous in space, providing the opportunity to use spatial heterogeneity to reduce risk. For example, degraded coral reefs that have shifted to algae-dominated could be recolonized by adjacent coral patches. Similarly, fishermen who spread their pressure on marine systems across space and let areas protected or closed for recovery are less likely to collapse their resources.
Authors
Juan-Carlos Rocha
References
Biggs, R., Carpenter, S. R. & Brock, W. A. Turning back from the brink: Detecting an impending regime shift in time to avert it. P Natl Acad Sci Usa 106, 826–831 (2009).
Rocha, J. C., Biggs, R. O. & Peterson, G. D. in Berkshire Encyclopaedia of Sustainability: Ecosystem Management and Sustainability (Craig, R. K., Nagle, J. C., Pardy, B., Schmitz, O. J. & Smith, W. K.) 5, 464 (Berkshire Publishing Group, 2012).
Scheffer, M. Critical Transitions in Nature and Society. (Princeton University Press, 2009).
Scheffer, M., Carpenter, S., Foley, J., Folke, C. & Walker, B. Catastrophic shifts in ecosystems. Nature 413, 591–596 (2001).
Carpenter, S. R. Regime shifts in lake ecosystems. (Ecology Institute, 2003).
Bellwood, D., Hughes, T., Folke, C. & Nyström, M. Confronting the coral reef crisis. Nature 429, 827–833 (2004).
Holling, C. S. Resilience and stability of ecological systems. Annual review of ecology and systematics 4, 1–23 (1973).
Lewontin, R. C. Meaning of Stability. Brookhaven Sym Biol 13–& (1969).
Folke, C. et al. Regime shifts, resilience, and biodiversity in ecosystem management. Annu Rev Ecol Evol S 35, 557–581 (2004).
Scheffer, M. & Carpenter, S. Catastrophic regime shifts in ecosystems: linking theory to observation. Trends in Ecology & Evolution 18, 648–656 (2003).
Scheffer, M. et al. Anticipating Critical Transitions. Science 338, 344–348 (2012).
Leave a Comment